Design of cathodic protection systems is essential to power plant operation
July 30, 2020 •Corrosion CONTROLLED, Corrosion Essentials, CP
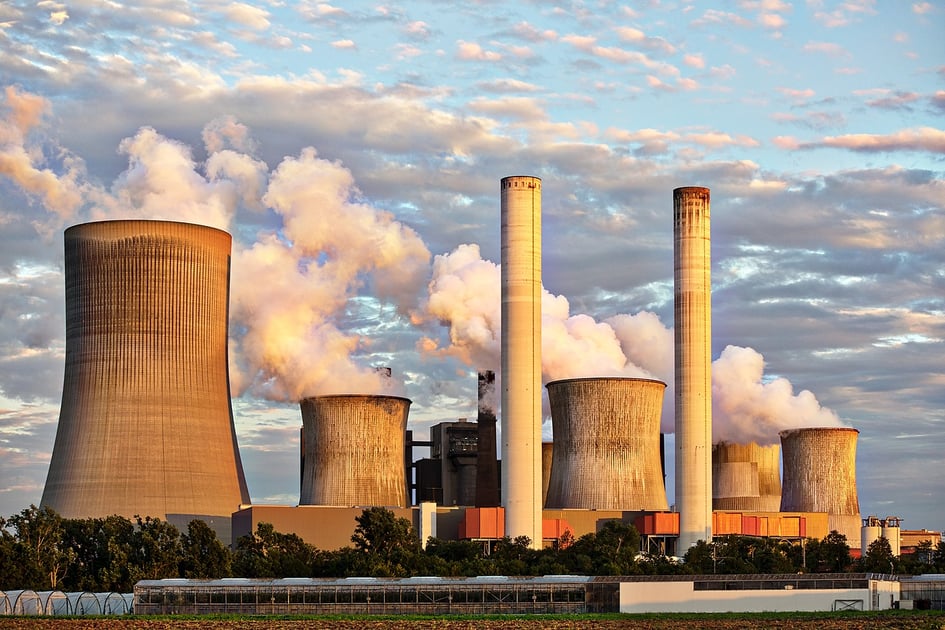
Designing cathodic protection (CP) systems for buried piping in power plants and other similar industrial facilities offers several unique challenges. The following discusses these challenges and provides case histories to illustrate the impact they have on CP system design, safety, and operation.
The types of anode systems available for cathodic protection system design fall into three configurations: shallow distributed groundbeds, deep anode groundbeds, and linear anodes. Each of these can be used in power plant piping CP systems either individually or in combination. Anode types fall into two categories: galvanic or impressed current. Both are used to protect power plant piping systems. As with most engineering challenges, each power plant application is unique and there is no single solution that can be applied universally.
Four principal considerations are crucial for any CP system in a power plant. First is the highly congested underground environment that is common to most power plants. The second critical factor is isolation in the presence of a pervasive copper grounding network. Third is the growing use of buried stainless steel (SS) piping. And finally, perhaps the most important and yet frequently overlooked issue is that of maintenance or the complete lack thereof.
Congested Underground Environment
Power plants and other similar industrial facilities typically have very congested underground environments. CP designers must consider the critical buried piping, but must also consider other structures having a significant impact on the CP system, i.e., copper grounding grids; steel of reinforced concrete piles; reinforced concrete foundations; ductile iron piping; storm drains and gravity-fed sewers; electrical duct banks; metallic fire hydrant risers with high-density polyethylene (HDPE) piping; and HDPE piping with metallic clamps.
The congested underground environment can adversely affect the CP system design. It can also add significantly to the current requirements and must be accounted for in the CP system design. These underground structures can shield current from the intended piping structures, creating current distribution problems. Also, the CP system can cause stray current-induced corrosion on unintended structures because of interference.
Electrical isolation is a major concern when engineers design a CP system for a power plant. While isolating a single pipeline segment from point A to point B can be achieved rather simply through the use of isolating flanges that the pipeline company regularly tests and maintains, the realities of power plant piping networks significantly complicate the practical expectation of isolation. By code, everything above grade in a power plant must be grounded, yet it is common to see CP systems designed based on isolation of the buried piping. Even if isolation is achieved during the plant construction, maintaining isolation over the life of the facility may not be realistic.
Given the speed with which these plants are erected, achieving isolation during construction is no simple task. Once installed, isolation flange kits require regular monitoring and periodic replacement that often does not occur. Piping modifications and other plant maintenance activities can also cause an inadvertent loss of isolation. Reliance on isolation over the life of the facility may drastically reduce the CP system design requirements, but at what cost should that isolation be lost?
Buried Stainless Steel Piping
NACE International Standard SP0169-2013 (formerly RP0169) Typical carbon steel (CS) piping CP applications are designed to ensure that a minimum threshold of current density (CD) (milliamps per square foot of surface area) is applied to the CS. CS piping systems can tolerate a wide range of over-polarization; at some point, excessive polarization can begin to cause coating failures and possibly hydrogen damage. With SS, however, too much current can actually cause piping failures, since the threshold for over-polarization is quite low. It is critical that the design of CP systems protecting SS piping meets the threshold minimum CD without over-polarizing any section of the piping system.
Maintenance
Finally, power plant CP systems must be designed with the full understanding that these systems may receive little or no maintenance for long periods of time. CP systems are not necessary to generate power. They are rarely hooked up to the plant’s data-control system and as such are usually not automatically monitored, unlike the regulated oil and gas pipeline industry.
Case History No. 1—3350-mW Grass Roots Gas Turbine Power Plant, Chicago, Illinois
Consisting of eight combustion turbines fueled by natural gas, a switchyard, a power distribution center building, and an operations building, this plant’s CP system was designed by the engineering, procurement, and construction (EPC) contractor’s in-house CP group. The group designed a series of nine deep anode groundbeds to protect all underground structures without isolating the piping from the balance of the plant, including the copper grounding network. The location of the groundbeds was set up to minimize the potential for interference with a third-party gas transmission line running parallel to and 5 ft (1.5 m) from the plant’s southern property line. The deep anode groundbeds were located along the northern and eastern edges of the plant’s property line. Unfortunately, during installation of the deep anode groundbeds, bedrock was hit at ~30.4 m so the anodes could not be installed to the 45.7-m depth as originally designed. The reduced depth, combined with the presence of a pervasive copper grid and the large foundations for the combustion turbines, created a significant current distribution problem with current not reaching the critical gas piping along the plant’s southwest corner. The solution for this facility was to install a second impressed current CP system, consisting of ~365.8 m of linear anode being trenched in parallel to the gas supply line. The trenching process caused some damage to the copper grounding grid, but this was easily repaired after the anode installation. The linear anode, located close to the gas pipeline, was able to quickly bring those sections of the pipeline back up to the appropriate NACE –850 mV polarization criteria level.
Case History No. 2—665-mW Simple Cycle Peaking Facility, Central Illinois
This power plant had a simple array of underground structures consisting of an incoming gas line, which ran along the plant’s southern edge and turned to the center of the plant where it came above ground to two gas treatment units. From the gas treatment units (A and B), a gas line ran east, feeding each of four simple cycle gas turbines, and a separate gas line ran west, feeding another four turbines. Each turbine unit had its own individual packaged cooling tower with buried cooling water supply and return lines running to the turbine. All cooling water lines were bonded to the gas lines and galvanic anodes were installed to protect each of the three isolated piping systems (incoming gas line, turbine group A gas and water piping, and turbine group B gas and water piping). A simpler galvanic underground piping CP system could not be found in a power plant. After reading an article on CP in an engineering magazine, the plant manager decided to have his plant’s CP system tested. The plant was approximately one year old and the EPC contractor who had built the plant did not or could not provide a commissioning report for the CP system; this left the plant manager concerned—hence his desire to have a third party test the system. During testing, the incoming gas line system and the group B gas and water piping were operating according to NACE criteria. The group A gas and water piping systems were not operating properly. The piping system had either lost isolation or never had proper isolation, as the galvanic anodes were rapidly discharging current directly to the copper grounding system with no appreciable effect on the underground piping. The EPC contractor was brought back to determine where isolation had been lost. If the plant manager had not read the article and contracted with a third party to test the CP system, the anodes on group A would have quickly depleted, leaving the gas and water piping vulnerable to corrosion.
Case History No. 3—1,180-mW Combined Cycle Generating Facility, Eastern Pennsylvania
This facility, consisting of two combined-cycle, natural gas-fired and steam-driven turbine units that generate ~590 mW each, was built on an existing brown field site that had formerly contained a large steel mill and associated operations. The soil suffered from years of chemical contamination and the entire power plant was built on steel piles. The piping system design was rather complex and the EPC contractor determined that isolation was not a practical consideration. The original CP requirements called for a system to protect all buried metallic structures. The projected current requirements exceeded 1,000 A, and current distribution issues posed significant challenges. The general contractor’s underground piping mechanical subcontractor was tasked with providing the CP and issued a package to bid. During the bid evaluation, an alternative proposal to protect only the buried piping was submitted using a localized linear anode system. The owner had no need to protect the steel piles because they had a significant corrosion allowance in their design. The linear anode-based system was accepted. This alternative significantly reduced the total current requirements from in excess of 1,000 A to A, including allowances for current losses to the copper grounding system and piles, while providing assurance that all buried piping received proper CP.
Find a certified Cathodic Protection specialist. Or, be one.
Source: “Designing Cathodic Protection for Power Plant Applications,” by Ted Huck, Vice President, Sales & Marketing, Matcor, Inc. Originally appeared on materialsperformance.com
FREE DOWNLOADS
White Paper: An Action Plan for Reducing Pipeline Failures, Costs with Corrosion in the Water Sector
Special Report: The Future of Corrosion Control, Insights from the Experts
Get Updates
Featured Articles
Categories
- 2024 Olympics (1)
- 2024 Water Resource Development Act (1)
- Abrasive Blasting (1)
- Advanced coating materials (9)
- Advanced Corrosion Control in Oil and Gas Industry (2)
- Advocacy (2)
- AI (2)
- Aircraft (1)
- Alkanization (1)
- AMPP (4)
- AMPP Annual Conference + Expo (2)
- Ampp Chapters (1)
- AMPP conference (1)
- AMPP logo (1)
- Ampp Membership (1)
- Ampp Standards (1)
- Amusement parks (4)
- Architectural (1)
- Architectural Coatings (2)
- Artificial Intelligence (1)
- Asset integrity (10)
- Asset maintenance (3)
- Asset Protection (1)
- Bim Software (1)
- Biodeterioration of materials (5)
- Biofouling (4)
- Blasting (1)
- Bridges (3)
- career development (1)
- cathodic protection (1)
- Cathodic Protection-CP (16)
- Ceramic epoxies (1)
- Certification (3)
- Chemical Injection (1)
- Civil Engineering (1)
- Coating inspector (1)
- Coating inspector jobs (1)
- Coating inspector program (1)
- Coatings (13)
- Coatings Application (2)
- Coatings failures (2)
- Coatings Industry (2)
- Coatings inspector (2)
- Coatings measurement and inspection (9)
- Coatings Systems (1)
- Cold stress (1)
- Concrete (12)
- Conference and Events (2)
- Corrosion (16)
- Corrosion Basics (5)
- Corrosion Control (15)
- Corrosion Control and Management (23)
- corrosion engineering (1)
- Corrosion Essentials (19)
- corrosion mitigation (1)
- Corrosion Prevention (6)
- Corrosion Under Insulation (1)
- cost of corrosion (1)
- Crevice Corrosion (1)
- Cui (1)
- Data Monitoring (1)
- Department of Defense (3)
- Deposition corrosion (1)
- Dissimilar Metal Corrosion (1)
- Dissolved gases (1)
- DoD (3)
- Education (2)
- Energy industry (9)
- entertainment industry (1)
- Epoxy (2)
- Fireproofing (1)
- Flexible coatings (2)
- Flint, Michigan (1)
- Fluoropolymer coating (3)
- Forms of Corrosion (4)
- Freshwater salinization (1)
- Galvanic (1)
- Galvanic Corrosion (3)
- General Corrosion (2)
- Hand tools (1)
- Industrial Application (3)
- Industrial Safety (2)
- Industry Best Practices (1)
- Industry Standards (1)
- infrastructure (2)
- Inspection (1)
- integrity management (1)
- Intergranular Corrosion (1)
- Intumescent Coatings (1)
- key note speaker (1)
- Machine Learning (1)
- Maintenance (2)
- Maritime Coatings (11)
- Maritime industry (11)
- Master Painters Institute (2)
- materials performance (1)
- Membership (2)
- Membership Benefits (2)
- Michio Kaku (1)
- Microbiological forms (1)
- Microbiologically influenced corrosion-MIC (11)
- Military (2)
- Mineral constituents (1)
- MPI (2)
- mpi training (1)
- Navy (1)
- Non-Destructive Testing (1)
- Oil and Gas (2)
- Oil Fields (1)
- Organic matter (1)
- Oxgen (1)
- Paint and Protective coatings (32)
- Paint specification (1)
- Personal Protective Equipment (3)
- Petrochemical Plant Fireproofing Methods (1)
- Petrochemical Plants (1)
- Pipeline (2)
- Pitting Corrosion (2)
- Pitting Detection (1)
- Power plant (1)
- Power tools (1)
- PPE (3)
- professional development (1)
- Protective Coatings (5)
- quality assurance (1)
- Real-Time Corrosion Monitoring in Oil Fields (1)
- Rebar Corrosion (1)
- rectifier (1)
- Reliability (1)
- Remote monitoring and drones (4)
- Repaint (1)
- Restoration (1)
- ride maintenance (1)
- Road deicers (1)
- Roads and bridges (1)
- Roller coaster (1)
- Rust (1)
- sacrificial anodes (1)
- Safety (5)
- Safety Standards (2)
- Salt pollution (1)
- Sensors (1)
- Ship Coatings (9)
- Shiptanks (1)
- Standards (9)
- Standards Committees (1)
- Steel (7)
- Steel Reinforcement (1)
- Stress Corrosion Cracking (1)
- Structural Steel (1)
- Surface Preparation (13)
- Sustainability (1)
- Sustainability and corrosion (7)
- Tools (1)
- Turbine (1)
- Types of Corrosion (1)
- Uniform Corrosion (1)
- us army core of engineers (1)
- Water crisis (1)
- Water pipe corrosion (1)
- Water quality (1)
- Water tank coatings (5)
- Water/treatment infrastructure (20)
- Waterway salinity (1)
- Workforce development (1)
- WRDA (1)